The methods used in the NUCLEON experiment
There are two methods in NUCLEON experiment used for measuring the energy of cosmic rays in the energy range 1012-1015 eV at the same time: a well-known technique of the ionization calorimeter and the newly developed KLEM (Kinematic LightweightEnergy Meter) method.
1. KLEM methodology and its implementation
A new method to determine the particle energy of the primary cosmic radiation for the study of cosmic rays in the energy range 1012-1015 eV has developed. It based on the measurement of the spatial density of secondary particles flux, produced in the first act of inelastic nuclear interaction in the plant target and the last layer of fine converter. The reproduction of electromagnetic component (g –quanta from the decay of neutral pions) comes in the converter. The method was the result of generalization of the well-known Castagnoli method (the measuring of angular characteristics of the secondary particle tracks, born in the first act of inelastic nuclear interaction in the target). The proposed method allows establishing of scientific equipment with relatively low weight and large transmission. Itsupposed to use first in the NUCLEON satellite experiment. The new method was tested on beams of SPS charged particles of accelerator at CERN. Test results confirmed the possibility of energy measurement by the proposed method, which allows to make a conclusion about the possibility of orbital experiment with the proposed equipment and solving the scientific problems.
1.1. GENERAL DESCRIPTION OF KLEM METHODS
KLEM methods - a new approach to energy measurement has been proposed, in order to promote direct studies of cosmic rays at higher energies. The highlight is the measurement of primary particle energy of the angular distribution of secondary particles flux, produced in a thin target in the first act of inelastic interactions and reproduction in a tungsten converter. The method makes it possible to create a scientific equipment with relatively small weight at high value of transmission and to conduct the “direct” research of CR at high energy in the range of 1014 - 1015 eV. The NUCLEON space experiment project has been proposed on the basis of KLEMmethods.The aim of this project is the research of CR in the area before the "knee" at the energy range of 1012 - 1015 eV. The most interesting area in this range is the 1013 - 1015 eV, here the available data are contradictory and do not have statistical reliability. The project was supported by the Russian Academy of Sciences andincluded in the Federal Space Program of Russia.
1.2. KLEM METHODOLOGY AND AIMS OF ACCELERATOR EXPERIMENTS.
The proposed method for determining the energy of cosmic ray particles is essentially a combination that includes both the measurement of kinematic characteristics (sensitive to the Lorentz factor of the primary particles) and fine inching installation (it measure the energy released in the electron-photon component).The usual kinematic method is based on the registration of angles emission of secondary particles produced in the act of particle inelastic interaction with the atomnucleus target. In this case the Lorentz factor of the incident particle g = E / m (value is directly related to the energy E and particle mass m) is determined by measuring the angles emission of secondary particles qi, and findingthe average valueof the tangent logarithm of these angles ln (E / m) ~ <-ln tg q>. The formula shows that the greater the particle energy, the smaller the averageangle emissionof secondary particles, and thusthe cascade becomes strait.In the case of KLEM methods tracks ofindividual particles in the studied event are not allocated, butthe spatial distribution of density of secondary particles are built, measured bymicrostrip detectors which have a spatial resolution of 100-500 microns. The detectors located at a distance H from the target, where is the top of the primary interaction (see Fig. 1). Spatial distribution characterized by the parameter S (E) = Σln2tgqi / 2, which is sensitive to both the angle emission of the particles and their multiplicity. The S parameter can be expressed for real experiment as S = Σln2 (2H / Xi) Ni, where Xiis the strip distance to the shower axis, Niis a total ionization, proportional to the number of singly charged particles caught in the strip.
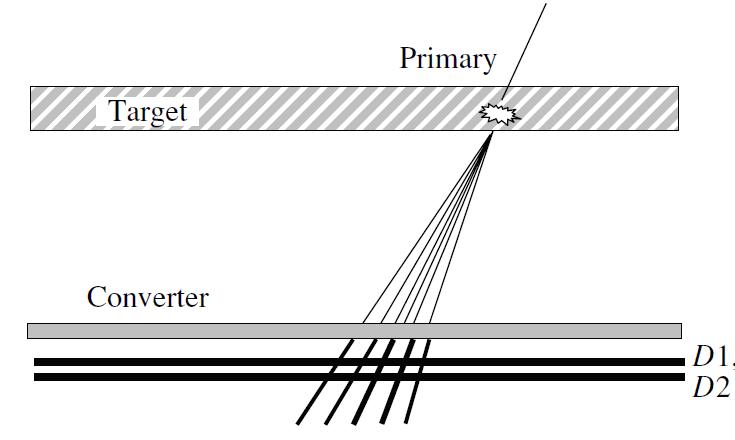
Fig.1.1. The scheme of KLEM methods
Fig.1.2 qualitatively shows the energy dependence of the distributions on pseudorapidity (in fact, on the angels) and the contribution of converter.
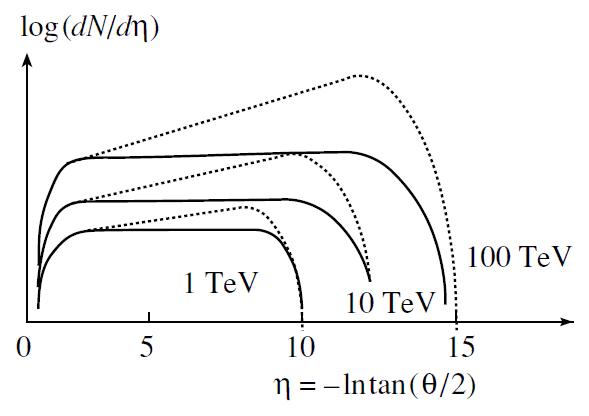
The feasibility of using the proposed method was evaluated for large-scale modeling [1] with the GEANT program complex 3.21 [3] with an additional QGSJET connected generator of nuclear interactions [4], which allows to describe the hadron-nucleus and nucleus-nucleus interactions at high energies. Various forms of spectrometer, operating at KLEM methodsat wide energy range,was studied. Simulations have shown that the KLEM method gives a power energy dependence of the parameter S ~ Eb in a wide range 1011-1016 eV for all types of nuclei (Z = 1-30), the exponent of power dependence is β = 0.74-0.82. The determining error of the energy E with individual energy measurement of the particle is sufficiently large σ (E) ~ 0.7¸0.9. However, as it shown in [1], the determining error is considerably smaller than about 50%when measuring the decreasing power spectrum of CR (F (E) ~ E-2.7), and as a result, this method can be used to measure the power spectrum of particles in a required range of energies.
This method makes it possible to establish a recording apparatus of a relatively small weight at a significant value of its transmission, opening the prospect of establishing the orbital spectrometer with a long exposure time and the prospect of CR research in a wide energy range with a single procedure. In addition, it is necessary to measure the charge and transit angle of particle for solving the physical issue,i.e. to trace its path in the chamber. As this takes place, it is necessary to distinguish the useful event from the particles with a threshold energy of 1 TeVwe interested in, from low-energy and out aperture background events. All this requires in-depth tests in accelerator experiments with extracted beams, which were conducted on nuclear and hadron beams of SPS accelerator in CERN. The first test of method had conducted on the extracted beam of pionsin 2002 and showed the fundamental possibility of implementing such an experiment [2]. According to the calculated calibration curve the recovery energies as the Erec = aS1 / β have been attributed and the lg (Erec / E) distribution have been built for each particle. Distributions on the recovered energy for the primary pion with an energy of 350 GeV shown in Figure 3. The data obtained by this method are next: the average measured energy was <Erec> = 334 GeV, the rms deviation s = 0.79, and in the case of <Erec>modeling = 350 GeV, s = 0.78. The distribution form is very sensitive to the kindof calibration curve, and its coincidence with the model testifies the correctness of the method not only at 350 GeV, but also at other energies
The method testing for determining the energy of particles on the accelerator beams of known energy with KLEM method had confirmed both the correctness of selected algorithm for energy determining and correct device operation of the prototype equipment.Available results let us to conclude the possibility of orbital experiment with the proposed equipment and solving the scientific problems.
Distributions on the recovered energy. Primary particle the pion, 350 GeV. Solid line is an experiment (<Erec> = 334 GeV, s = 0.79), the dotted line is modeling (<Erec> = 350 GeV, s = 0.78).
2. Ionizingmicrocalorimeter
2.1. THE PURPOSE OF MICROCALORIMETER USE
Methods of ionization calorimeter is the most versatile and allows measure the energy and the electromagnetic and hadron components. The long experience in the application of this method allows use it for the flight calibration of the newly proposed KLEM method. The calorimeter partitioning gives the possibility to divide hadron and electromagnetic cascades on the spatial distribution of ionization, what is necessary forresearch of CR electronic components research.
2.2. MICROCALORIMETER CALIBRATION
The energy release in the calorimeter is determined by using a recovery cascade curves. The correction factors are determined with the use of mathematical modeling. The installation testing was conducted on electron beams with different energies. Calibration curves shown on Fig. 2.1.
Fig.2.1. Calibration curvesfor microcalorimeter
There were two approaches to allow for the removal of energy share. In the first case,the approximation of the cascade curve was used, in the second the total energy was being multiplied by a constant correction factor. The fig.2.2 shows the distribution on recovered energy for electrons (the true energy of 100, 150, 200 GeV) in comparison with results of the simulation (dotted line).
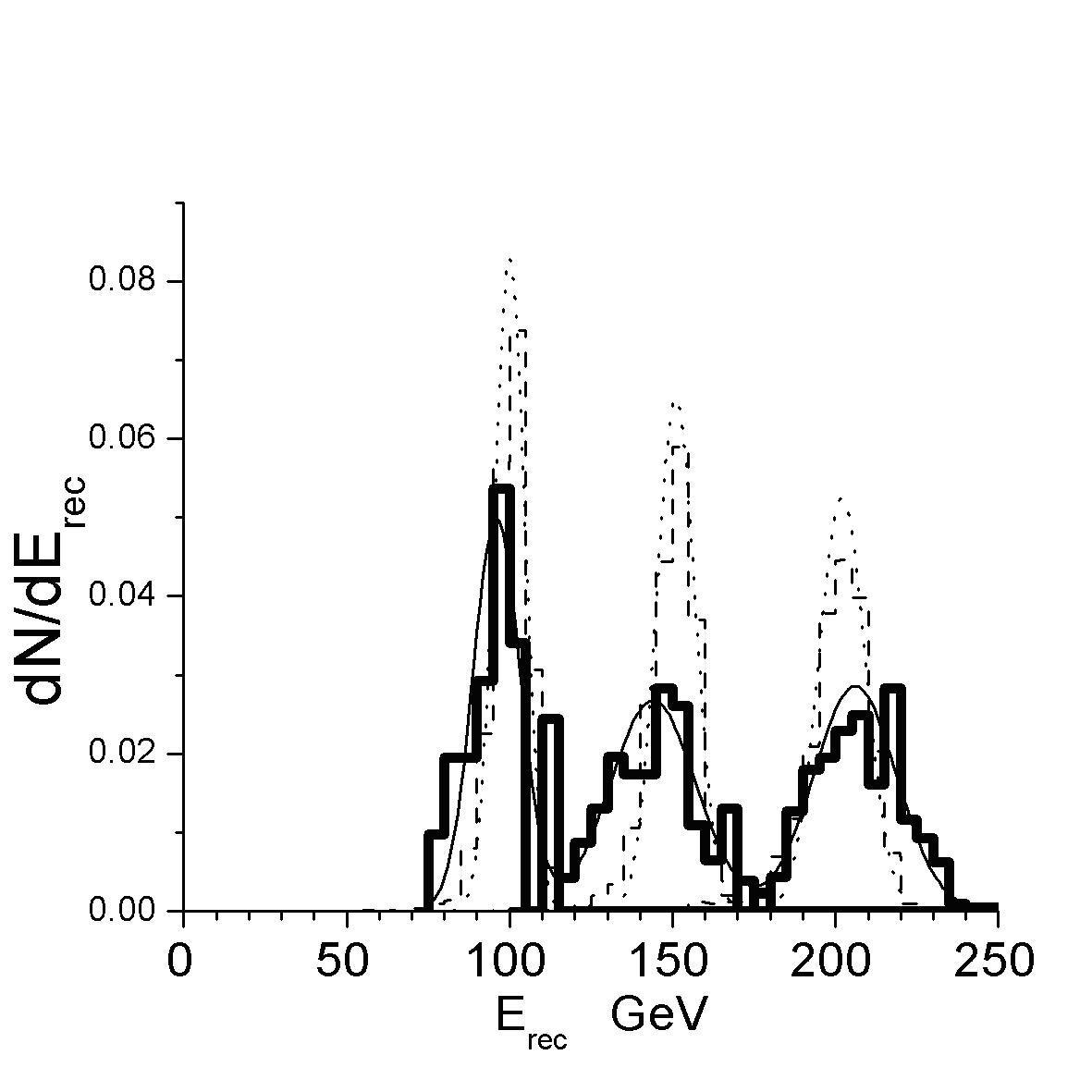
Fig. 2.2. Distributions on the recovered energy
(Bold is the experiment, dashed is modeling, thin solid is Gaussian approximation).
For electromagnetic event it’s better 10% resolution and it is 7.5%, 9.3%, 6.2% for the three beam energies used in the experiment (simulation gives 4.5%, 4.0%, 3.7% respectively). The particle trajectories recovers according to microstrip detectors of calorimeter and SEI, and the scintillator trigger system. The angular distribution reconstructed for pion beams with an energy of 300 GeV, is shown on fig.2.3. The angular accuracy is ~ 0.9 °.
Fig.2.3. The angular distribution of the pion beams
The calorimeter will be used for the separation of the electromagnetic and hadron components apart from the energy measurement. The cascade curve form depends on the nature of the primary particle. The accelerator testinghas confirmed the correctness of assumptions.
BIBLIOGRAPHY
1. Korotkova N.A., Podorozhniy D.M., Postnikov E.B. et al. // NP. 2002. V.65. №5. P.884
2. Bashinjaghyan G.L., Voronin A.G., Golubkov S.A. et al. // PTE. 2005. V.48. №1. P.46
3. GEANT User's Guide. CERN DD/EE/83/1 (Geneva), 1983.
4. Kalmykov N.N., Ostapchenko S.S., Preprint № 98-36 / 537, SINP MSU (Moscow, 1998).
5. Kalmykov N.N., Ostapchenko S.S., Pavlov A.I. // Nucl. Phys. B (Proc. Suppl.). 1997. V.52B. P.17.